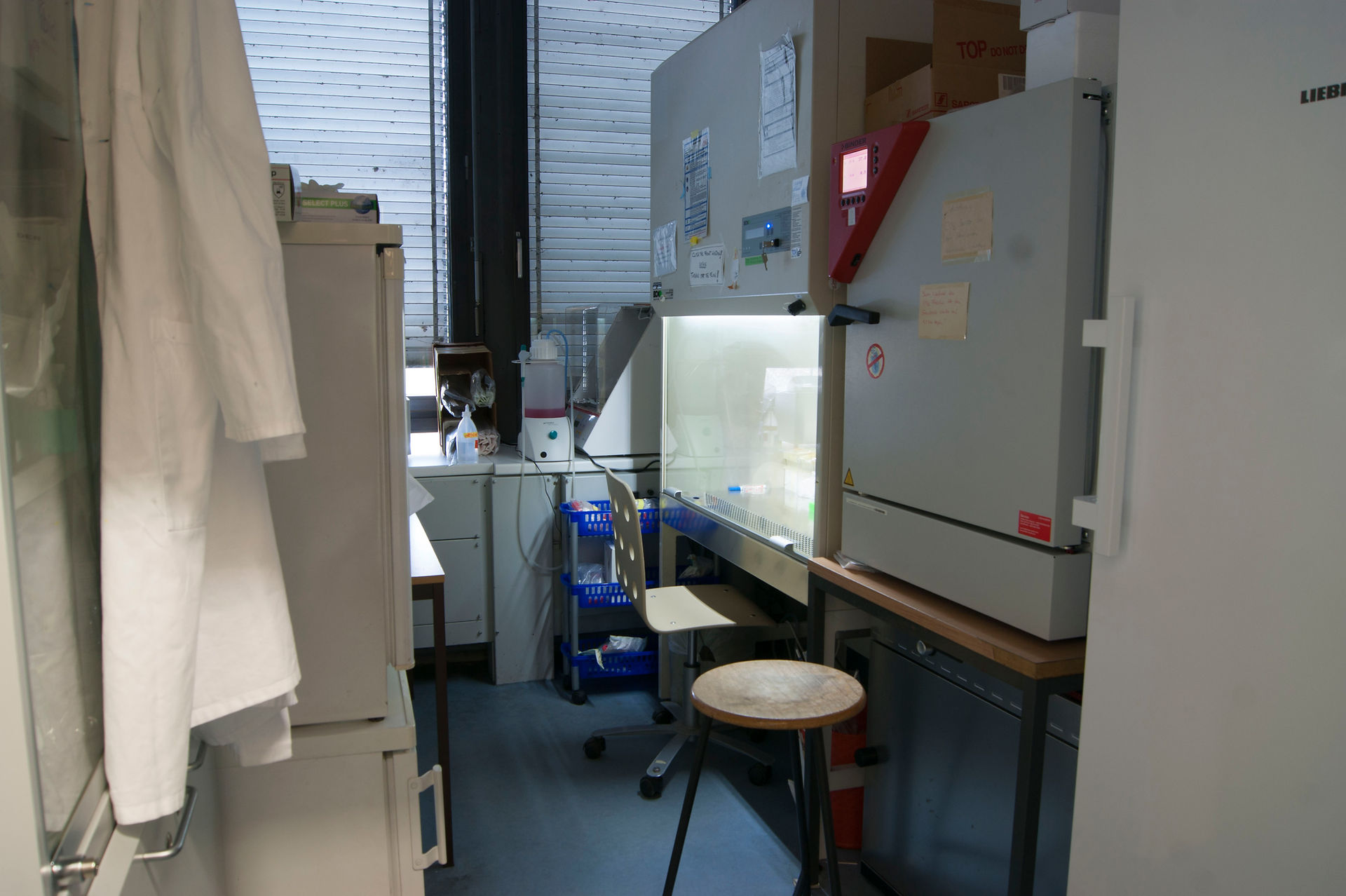
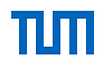
Epithelial branch elongation is a central developmental process during branching morphogenesis in diverse organs. This fundamental growth process into large arborized epithelial networks is accompanied by structural reorganization of the surrounding extracellular matrix (ECM), well beyond its mechanical linear response regime. Here, we report that epithelial ductal elongation within human mammary organoid branches relies on the non-linear and plastic mechanical response of the surrounding collagen. Specifically, we demonstrate that collective back-and-forth motion of cells within the branches generates tension that is strong enough to induce a plastic reorganization of the surrounding collagen network which results in the formation of mechanically stable collagen cages. Such matrix encasing in turn directs further tension generation, branch outgrowth and plastic deformation of the matrix. The identified mechanical tension equilibrium sets a framework to understand how mechanical cues can direct ductal branch elongation.
B. Buchmann, L. K. Engelbrecht, P. Fernandez, F. P. Hutterer, M. K. Raich, C. H. Scheel A.R. Bausch (2021)
Mechanical plasticity of collagen directs branch elongation in human mammary gland organoids
Nature Communications, 12, 2759
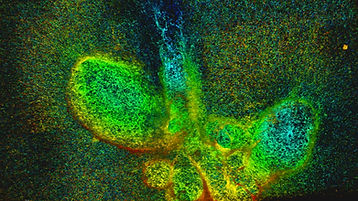

Orientation of the division axis can determine cell fate in the presence of morphogenetic gradients. Understanding how mitotic cells integrate directional cues is therefore an important question in embryogenesis. Here, we investigate the effect of dynamic shear forces on confined mitotic cells. We found that human epithelial cells (hTERT-RPE1) as well as MC3T3 osteoblasts align their mitotic spindle perpendicular to the external force. Spindle orientation appears to be a consequence of cell elongation along the zero-force direction in response to the dynamic shear. This process is a nonlinear response to the strain amplitude, requires actomyosin activity and correlates with redistribution of myosin II. Mechanosteered cells divide normally, suggesting that this mechanism is compatible with biological functions.
P. Fernandez, M. Maier, M. Lindauer, C. Kuffer, Z. Storchova, A.R. Bausch (2011)
Mitotic Spindle Orients Perpendicular to the Forces Imposed by Dynamic Shear
PLOSone, 6, e28965
To understand mechanotransduction, purely mechanical phenomena resulting from the crosstalk between contractile cells and their elastic surroundings must be distinguished from adaptive responses to mechanical cues. Here, we revisit the compaction of freely suspended collagengels by embedded cells, where a small volume fraction of cells (osteoblasts and fibroblasts) compacts the surrounding matrix by two orders of magnitude. Combining micropatterning with time-lapse strain mapping, we find gel compaction to be crucially determined by mechanical aspects of the surrounding matrix. First, it is a boundary effect: the compaction propagates from the edges of the matrix into the bulk. Second, the stress imposed by the cells irreversibly compacts the matrix and renders it anisotropic as a consequence of its nonlinear mechanics and the boundary conditions. Third, cell polarization and alignment follow in time and seem to be a consequence of gel compaction, at odds with current mechanosensing conceptions. Finally, our observation of a threshold cell density shows gel compaction to be a cooperative effect, revealing a mechanical interaction between cells through the matrix. The intricate interplay between cell contractility and surrounding matrix mechanics provides an important organizing principle with implications for many physiological processes such as tissue development.
P. Fernandez, A.R. Bausch (2009)
The compaction of gels by cells: a case of collective mechanical activity
Integrative Biology, 1, 252-259
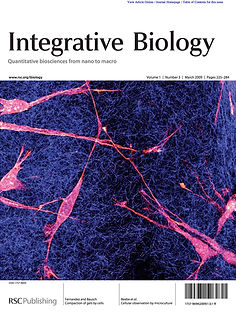